Wearable medical devices and implants are becoming more prominent in a wide range of applications such as health monitoring devices and biomedical implants, which provide continuous measurements of biomarkers and medical diagnostics. Based on growth of the elderly population and insights garnered from the pandemic, the shift to the development of wearables and biosensors has increased the need for real-time health monitoring, personalized medicine and point-of-care technology (POCT).
Over the last decade, advancements have been made in the development of next-gen medical wearables and implants using the latest technologies that make using them cheap and readily available. What typically took hours for diagnostic testing at a medical facility can now be done remotely using biosensors. As more healthcare services shift towards personalized medicine, those sensors will become as commonplace as Fitbits and smartwatches. According to a report from Facts & Factors, the wearable technology market will surpass $380 billion by 2028 with a compound annual growth rate of 18.5%.
Wearables and sensor types
So what is wearable medical technology? Most of us have seen or used devices that monitor and collect a number of health vitals and exercise regimens and can even send that data to health professionals in real time. These can be fitness trackers and smartwatches, but others, such as wearable ECG monitors and blood pressure sensors, are not so common. Withing’s Move ECG device, for example, can measure an electrocardiogram and even detect atrial fibrillation, then send data to the user’s doctor.
In 2019, Omron Health launched its HeartGuide, a wearable oscillometric blood pressure monitor. The watch can collect and store up to 100 readings, which can be transferred to the HeartAdvisor mobile app for review, comparison and treatment optimization. Biosensors are also becoming more readily available and can be worn on the skin or even as implants or used in a smart-pill form. Philips’ wearable biosensor, for example, is a self-adhesive patch worn on the skin and provides several biometrics, including movement, heart rate, respiratory rate and blood pressure.
Biosensors can be traced back to 1962 when Leland Clark designed a glucose sensor based on an oxygen electrode, which was used during cardiovascular surgeries. Since then, many advancements have been made within the biosensor field as new technologies have become available. Biosensors are bio-analytical devices that provide specific quantitative and semi-quantitative analytical information by converting biological reactions or stimuli into measurable signals.
Those sensors are comprised of three key components: a biological sensing component, a connected detector or transducer component, and a signal processing system. That bio component can be an enzyme, antibody, cell or many others, while the transducer is based on the transduction method, such as electrochemical, optical, acoustic or calorimetric. In a biomedical context, biosensors need specific requirements before they can be used internally, with the first being biocompatibility. Sensors in skin integration applications, for example, need to be stretchable, flexible and ultra-thin to conform to the natural flex and movement of the user.
To that end, there are factors that present problems in developing medical wearables and biosensors, especially those that are implantable. On the top of that list is the power source, as even those used internally require batteries, which tend to be large and bulky for sensors designed for long-term use. The good news is that scientists and researchers have begun to develop power systems that make use of energy-harvesting technologies, which could power those biosensors almost indefinitely.
Energy harvesting types and techniques
One of the significant challenges with battery-operated medical devices is autonomy. An increasing number will require greater battery life and power, but internal devices have space constraints, and larger batteries are not feasible. With new advancements, those bulky batteries can be replaced using energy-harvesting technologies. Wearables and biosensors are being designed that only require a small amount of current to detect, store and transmit vital functions, and energy harvesting can supply enough power for them to function for long periods.
There are many harvesting methods that can be employed for those medical devices depending on the application. One is the triboelectric effect, which produces surface charges on a pair of dissimilar materials when they contact and separate. These systems require a minimum number of components: two layers of triboelectric material, physical separation between them, and electrodes for collecting electricity. Others include thermal energy harvesting, vibrational energy, RF energy, motion energy and even the incorporation of supercapacitors to store that energy when the user isn’t engaged in any activity.
Examples of medical wearables and implantable biosensors using energy harvesting
Researchers have developed novel solutions to power medical wearables and implantable devices using energy-harvesting techniques. Researchers at the University of Massachusetts have developed a microbial biofilm as a flexible material worn on the skin that’s powered by sweat. The single sheet is about 40 µm thick and is produced using an engineered version of the Geobacter sulfurreducens bacteria.
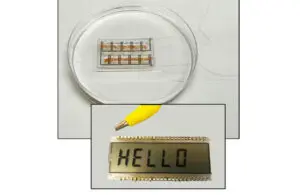
The UMass biofilm uses bacteria to generate power via the wearer’s sweat and can power small LCDs. [Image courtesy of UMass]
The flexible sheet harvests energy using bacteria colonies with individual microbes connected via a series of natural nanowires. A team then laser etches small circuits into the film, sandwiches them between electrodes and seals them a breathable, soft, sticky polymer. The film produces enough energy as the user’s sweat evaporates to power a small LCD, although the researchers are looking at ways to expand that power to drive electronics and other wearables.
Ohio State University scientists have also harnessed sweat’s power using a biosensor Smart Necklace. The wearable sensor detects glucose levels excreted from the skin during exercise. The sensor uses a resonance circuit that reflects RF signals sent out by an external reader system. The coupling of the sensor interface and an inductor-capacitor oscillator via a pair of varactor diodes converts a change in electric potential into a modulation of the capacitance, resulting in a shift of the resonant circuit. The necklace only requires a minimal amount of sweat to function and has the potential to detect other biomarkers secreted through the skin.
Engineers at Texas A&M University have developed a device that uses graphene and injects AC current into the skin to monitor blood pressure. Dubbed graphene electronic tattoos, the array of stick-on graphene sensors can track cardiovascular health via continuous monitoring that garners blood pressure measurements during various situations, including exercising, sleeping and high-stress situations. The tattoos function by injecting AC current into the wearer’s skin and then analyzing the response to determine bioimpedance. By measuring blood pressure levels over hours rather than once or twice daily, healthcare professionals can eliminate any noise in the data, which provides a more accurate picture of the user’s blood pressure.
Scientists at Georgia Tech University developed a small wireless sensor that can be implanted in the brain’s blood vessels to monitor the healing of aneurysms. The sensor operates without batteries and can be wrapped around stents or diverters implanted to control blood flow. The sensor is manufactured using aerosol jet 3D printing to produce conductive silver traces on elastomeric substrates. The sensors are inserted via a catheter and use the inductive coupling of signals to allow wireless detection of biomimetic cerebral aneurysm hemodynamics. A coil picks up electromagnetic energy transmitted from another coil located outside the body. When blood flows through the stent, the implanted sensor changes its capacitance and alters the signals passing through the sensor to a third coil outside the body.
Engineers at the Massachusetts Institute of Technology have developed a novel battery powered by glucose that measures just 400 nanometers thick, or about 1/100th of the diameter of human hair. The battery (pictured at the top of this post) can generate about 43 microwatts per square centimeter of electricity and withstand temperatures up to 600 ° C. This means it can handle the high-temperature sterilization process of most implantable devices. The battery was designed using an ultra-thin ceramic substrate and a glucose solution, which also provides a level of flexibility, making it easy to place within the body.
The battery has a three-layer design using the top as an anode, an electrolyte in the middle and a bottom that acts as a cathode. The anode reacts with glucose, transforming the sugar into gluconic acid and releasing a pair of protons and electrons. The middle electrolyte acts as a separator for the protons and electrons, conducting the protons through a fuel cell, where they combine with air to produce harmless water. The bottom layer provides electrical connectivity, making the sugar battery safe for operation within the body.
These are just a few of the advancements made to harness power for wearable and implantable medical devices. As newer technology becomes available, innovative medical designs will undoubtedly follow, making energy-harvesting devices more efficient with increased power. By incorporating the most accurate sensors into that technology, engineers could provide users with the most advanced healthcare monitoring. It could also have the potential to revolutionize healthcare by enabling patients to take a more proactive approach to their health and well-being.
Article Source:MDO